The availability of synchrotron radiation for research in geoscience opens new experimental possibilities by overcoming the former limitations such as detection limit and spatial resolution. Our group uses a broad energetic range of synchrotron radiation from high-energetic X-rays down to low-energetic infrared radiation for research mostly in-situ at high pressures in diamond anvil cells (DAC).
The very high brilliance X-rays available at the modern synchrotron sources, such as PETRA III at DESY in Hamburg, allows us to determine (by measuring X-ray diffraction, XRD) the compression behavior of geomaterials compressed in the diamond anvil cell (DAC) up to very high pressures relevant for the deep interior of the Earth. We are currently performing studies of the pressure-volume (P-V) equation of state of hercynite (FeAlO4) the iron end-member of the upper mantle peridotitic spinel and of the 3.65 Å phase, MgSi(OH)6 the product of decomposition of the 10 Å phase and a relevant candidate for the deep transport of hydrogen in the mantle through subduction. Moreover we examine the synthesis and properties of new materials containing Mg-Fe-N and Si-Ge.
Contact
Dr. Sergio Speziale
Dr. Hans Josef Reichmann
The coesite-stishovite transition of hydrous, Al-bearing SiO2
The P-T conditions of the transition of the silica polymorphs coesite (Coe) – stishovite (Sti) have been investigated in numerous experimental studies in the pure SiO2-system (e.g., Ono et al., 2017). However, Coe and Sti can incorporate significant amounts of Al and H in their structures by various substitution mechanisms. In this project we examine the influence of Al2O3 and H2O on the position of the Coe-Sti transition at P and T using the multi-anvil press at the synchrotron PETRA III, Hamburg, combined with in situ X‑ray diffraction measurements. As starting material, we use 100 % Sti which was formed in situ at 9 GPa and 900 °C prior to our equilibrium experiments from a mixture of amorphous SiO2 plus 1 wt % γ-Al2O3 plus 10 wt % H2O (filled in X-ray transparent Titanium-capsules).
![Example of synchrotron energy dispersive XRD pattern taken in situ at 8 GPa and 1400°C. At this conditions stishovite transforms with time into coesite. Reflections labelled Ti and MgO are from the Titanium-Capsule and the pressure transmitting medium, respectively.[Translate to English:] Beispiel eines energiedispersen XRD-Spektrums, aufgenommen am Synchrotron in situ bei 8 GPa und 1400°C. Unter diesen Bedingungen wandelt sich Stishovit mit der Zeit in Coesit um. Die mit Ti und MgO gekennzeichneten Reflexe stammen von der Titankapsel bzw. dem druckübertragenden Medium.](/fileadmin/_processed_/a/5/csm_Coesite-Stishovite-transition_Grafik_MKM_cbf9040ee0.jpeg)
Our preliminary results indicate that the transition is shifted to lower pressures compared to the pure SiO2 system (Ono et al. 2017). This is in accordance to the findings of Bolfan-Casanova et al. (2009) for the stishovite – post-stishovite transition, who observed a shift of the transition pressure from 80 GPa to 30 GPa at 2500 K by incorporation 3 wt% Al2O3 into stishovite.
Bolfan-Casanova, N., Andrault, D., Amiguet, E., and Guignot, N.: Equation of state and post-stishovite transformation of Al-bearing silica up to 100 GPa and 3000 K, Phys. Earth Planet. Inter., 174 (1-4), 70-77, https://doi.org/10.1016/j.pepi.2008.06.024, 2009.
Ono, S., Kikegawa, T., Higo, Y., and Tange, Y.: Precise determination of the phase boundary between coesite and stishovite in SiO2, Phys. Earth Planet. Inter., 264, 1-6, http://dx.doi.org/10.1016/j.pepi.2017.01.003, 2017.
Contact
Prof. Monika Koch-Müller
Dr. Christian Lathe
Dr. Bernd Wunder
Partner
Dr. Robert Farla, Deutsches Elektronen-Synchrotron DESY, PETRA III Extension
Breakdown of lawsonite to garnet, kyanite, coesite and H2O: kinetics, reaction mechanism, P/T stability
Lawsonite (CaAl2Si2O7(OH)2·H2O) is an important rockforming mineral containing 11.5 wt% H2O in its crystal structure. It occurs in blueschists and eclogites and therefore its dehydration plays an important role in subduction slabs. The most important dehydration reaction of lawsonite is its breakdown to garnet, kyanite, coesite and H2O in the pressure/temperature ranges 4 – 8 GPa/800 – 1000°C. However, the location of the reaction in the P/T space is not well constrained because of the limited dataset available in this range. Thus, the aim of this study is to fill these gaps in our knowledge by performing HP/HT experiments using the multi-anvil press at the synchrotron PETRA III, Hamburg, combined with in situ X‑ray diffraction measurements. Starting material is very pure natural lawsonite filled with water in excess into X-ray transparent Ti-capsules.
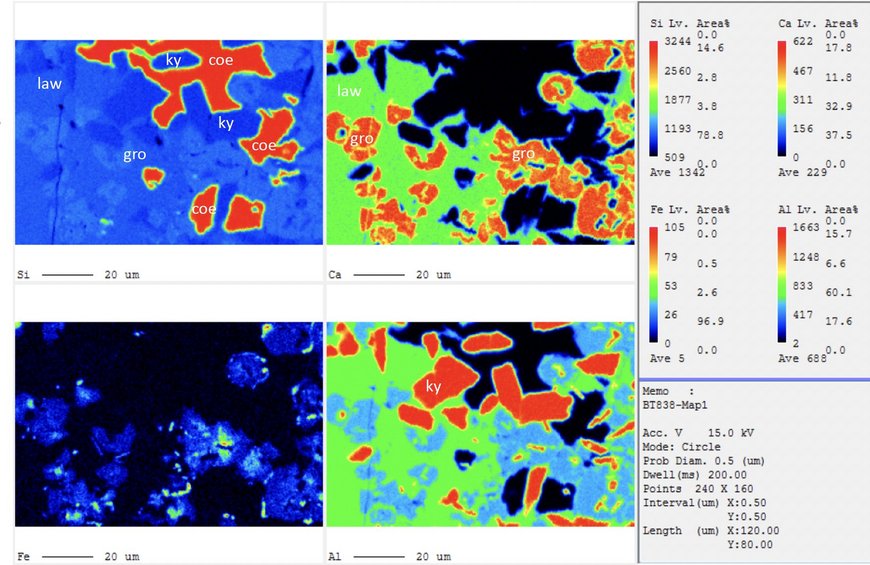
Contact
Prof. Monika Koch-Müller
Dr. Christian Lathe
Partner
Dr. Melanie Sieber, Universität Potsdam
Dr. Robert Farla, Deutsches Elektronen-Synchrotron DESY, PETRA III Extension
X-ray fluorescence
X-ray fluorescence (XRF) is the emission of characteristic "secondary" (or fluorescent) X-rays from material that has been excited by exposure to high-energy X-rays. The phenomenon is widely used for elemental and chemical analysis. Due to the high penetration depth of hard X-rays in matter, XRF can be used to determine element concentrations in complex sample environments, such as high-pressure cells. The high brilliance of synchrotron radiation strongly improves sensitivity and spatial resolution of XRF analyses and allows sampling of trace elements in materials that cannot be quenched to ambient conditions, such as aqueous fluids.
Contact
Dr. Christian Schmidt
Partner
Prof. Dr. Max Wilke, Universität Potsdam
X-ray absorption spectroscopy (XANES, EXAFS)
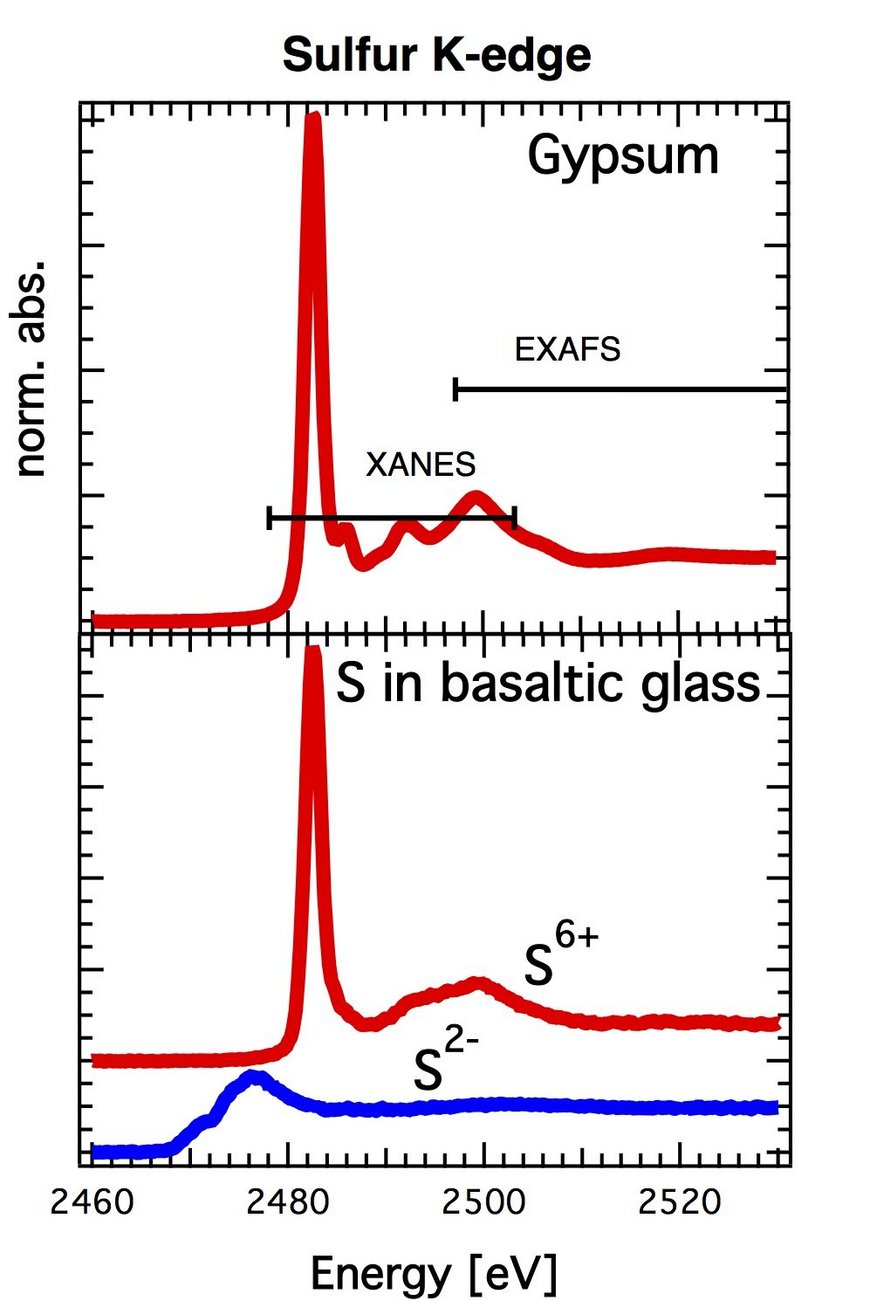
X-ray absorption spectroscopy uses x-rays to probe the physical and chemical structure of matter on the atomic scale. X-ray absorption spectroscopy is element-specific, which means that X-rays are chosen to be at and above the binding energy of a particular core electronic level of a specific element. Because most elements have core-level binding energies in the X-ray regime, nearly all elements except the lightest can be studied with this technique. The fine structure observed in the X-ray absorption is due to interaction of the photo-electron with unoccupied electronic states of the absorbing element or due to backscattering from neighboring atoms. The fine structure is usually divided into two regions: XANES, X-ray Absorption Near Edge Structure: Fine structure observed close to the binding energy, which is assigned to excitation of the photo-electron to localized states or to multiple backscattering of the photo-electron by neighboring atoms. The XANES region is sensitive to oxidation state, coordination and site geometry of the absorbing element. EXAFS, Extended X-ray Absorption Fine Structure: Fine structure observed at energies far above the binding energy, which is assigned to single backscattering of the photoelectron by neighboring atoms. Analysis allows determination of number of neighbors and distances to neighbors of the absorbing element in crystalline and non-crystalline samples. Both, XANES and EXAFS, are applicable to concentrated and dilute samples. Due to the high penetration depth of hard X-rays, measurements can be performed even at in-situ conditions using complex sample environments, such as pressure cells or reaction cells, at least for absorption edges above ca. 6 keV.
X-ray Raman scattering (XRS)
X-ray Raman scattering (XRS) is an inelastic x-ray scattering process, in which a high-energy X-ray photon transfers energy to a core electron, exciting it to an unoccupied state. The process is in principle analogous to X-ray absorption, but the energy transfer plays the role of the X-ray photon energy absorbed in X-ray absorption. Because the energy of the probing X-ray can be chosen in the hard X-ray regime, certain constraints of soft X-rays are overcome. For example, soft X-ray studies usually require vacuum environment. This makes X-ray absorption studies of samples that are not stable in vacuum, such as many liquids, impossible. One of the most exciting applications of X-ray Raman scattering is the study of soft X-ray absorption edges at high pressure. The employed high-energy X-rays easily pass through a high-pressure apparatus like a diamond anvil cell and reach the sample inside the cell.